In modern medicine, tissue selectivity – the ability of a hormone or drug to act on specific tissues – has become a cornerstone of advanced hormone therapy. This approach is exemplified by selective receptor modulators, such as Selective Androgen Receptor Modulators (SARMs), which are engineered to deliver targeted hormonal effects in certain tissues (like muscle and bone) while sparing others. By conferring high receptor specificity and tissue-specific actions, tissue selectivity in hormone therapy allows treatments to maximize benefits (for example, muscle growth or bone strengthening) with minimal hormonal side effects. In essence, scientists have transformed hormone therapy from a blunt systemic treatment into a form of targeted therapy, leveraging selective receptor modulation to achieve unprecedented pharmacological precision in endocrine treatment.
Traditional hormone treatments before the era of selectivity lacked this precision. For decades, therapies like anabolic steroids (synthetic testosterone derivatives) bathed the entire body in hormones, stimulating receptors in virtually all tissues. This yielded broad androgenic effects alongside the desired anabolic benefits – an indiscriminate approach that often caused as much harm as good. While muscles might grow, so would other tissues: the prostate could enlarge, skin glands would overproduce oil, and other organs could be overstimulated. Patients on early hormone regimens frequently experienced virilization, organ enlargement, metabolic issues, and a host of other side effects due to this one-size-fits-all dosing. These limitations made it clear that a new strategy was needed. Researchers recognized that if a drug’s action could be tailored to specific tissues, it might be possible to separate the positive effects of hormone therapy from the negative ones. This realization set the stage for a revolutionary approach – one that would make tissue selectivity in hormone therapy the central goal, paving the way for hormone therapy advancements that offer safer and more effective treatments.
Historical Background: Hormone Therapy Before Tissue Selectivity
Before tissue selectivity became a guiding principle, hormone therapies were powerful but imprecise tools. Early endocrine treatments relied on administering hormones or analogues that acted everywhere in the body. For example, testosterone therapy could restore muscle mass or libido in deficient patients, but it also activated androgen receptors in the prostate, liver, skin, and brain. Patients often faced hormonal side effects as a trade-off for benefits – men using high-dose testosterone might develop acne, hair loss, or prostate enlargement, while women could experience unwanted virilization (development of male characteristics). Estrogen treatments had a similar problem: while estrogen could strengthen bones or manage menopausal symptoms, it would also stimulate breast and uterine tissue, sometimes increasing cancer risks. In short, the concept of tissue-specific effects was largely absent in early hormone therapy, and physicians had to accept a wide gamut of effects, both good and bad.
Researchers did make some attempts to improve the balance of anabolic vs. side effects with traditional chemistry. Certain anabolic steroid modifications showed mild tissue preference – for instance, the synthetic androgen nandrolone causes slightly less prostate stimulation than testosterone because it is less readily converted to the ultra-potent dihydrotestosterone (DHT) in the prostate. Similarly, altering steroid molecules (e.g. 17α-alkylation of testosterone) enabled oral dosing but introduced new toxicities like liver damage. Despite these tweaks, a truly selective hormone drug remained elusive. Steroid hormones by nature circulated broadly and influenced multiple organs. This meant that therapeutic precision was practically impossible: one could not fully disentangle the desirable anabolic effects (such as muscle building or bone strengthening) from the undesirable effects (such as prostate growth, breast tissue stimulation, or cardiovascular strain). The lack of tissue selectivity kept hormone therapy something of a double-edged sword – effective, but with significant caveats.
By the mid-20th century, it became evident that a deeper scientific breakthrough was needed to solve this problem. The discovery of hormone receptors in the 1960s and 1970s provided a crucial insight. Scientists like Dr. Elwood Jensen demonstrated that hormones exert their effects by binding to specific receptor proteins in target cells. For example, estrogen receptors were identified in various tissues, and the androgen receptor (AR) was recognized as the key protein that testosterone activates. This shift – from viewing hormones as free-roaming signals to understanding the receptor “locks” they fit into – was transformative. Researchers realized that the key to selectivity might lie in the receptors themselves. If a drug could be designed to interact with a hormone receptor in just the right way, perhaps it could trigger only a subset of the receptor’s actions. In theory, one might turn on the muscle-building genes without also turning on, say, the prostate-growth genes. This idea set the foundation for future scientific breakthroughs in hormone therapy.
Scientific Breakthroughs of the 1980s: Foundations of Tissue Selectivity
The 1980s were a turning point that provided the first proof that tissue-selective hormone action was achievable – a true pharmacological innovation for endocrinology. A major breakthrough came from the field of estrogen research. Oncologists and pharmacologists developed Selective Estrogen Receptor Modulators (SERMs), with tamoxifen being the landmark example. Tamoxifen was designed in the 1960s as an anti-estrogen to treat breast cancer, but by the 1980s hormone research had revealed a curious phenomenon: tamoxifen could block estrogen’s effects in breast tissue(helping to starve estrogen-sensitive tumors) while mimicking estrogen in other tissues like bone and the uterus. In fact, studies in the 1980s showed tamoxifen prevented bone loss even as it acted as an estrogen antagonist in breast tissue. This was a radical concept at the time – a single drug acting as an estrogen antagonist in one tissue and an agonist in another. Scientists coined the term “Selective Estrogen Receptor Modulator” to describe this dual behavior. The discovery of tissue selectivity through tamoxifen was crucial for hormone therapy because it introduced a new paradigm: one could selectively modulate a hormone receptor to get different outcomes in different tissues. In other words, it proved that hormones no longer had to be all-or-nothing in their effects. This foundational discovery suggested that smarter, targeted hormone therapies were possible, overturning the old assumption that a hormone drug must invariably act the same way everywhere.
Several scientific advances in the 1980s underpinned this new paradigm. Molecular biologists found that when a compound like tamoxifen binds to a receptor, it can change the receptor’s shape subtly. These shape changes determine which co-regulator proteins inside the cell will attach to the receptor. Different tissues have different sets of these co-regulators. So, the tamoxifen-bound estrogen receptor could turn gene programs on or off in a tissue-dependent manner – activating bone-preserving genes in bone cells, for example, while blocking growth signals in breast cells. This mechanism explained how selective modulation worked at the molecular level. Such insights were a scientific breakthrough, revealing that a drug could “tune” a receptor’s activity rather than simply turning it completely on or off.
By the late 1980s, the success of SERMs had researchers asking: could the same strategy be applied to other hormones? Attention turned to the androgen receptor, which mediates testosterone’s effects. Traditional androgen therapy at that time still caused the familiar spectrum of anabolic and androgenic effects. But inspired by the SERM model, scientists imagined a “tamoxifen for androgens” – a compound that could build muscle and bone without the downsides of testosterone. Early clues suggested this was achievable. Notably, the first nonsteroidal anti-androgens (like flutamide, introduced in the 1980s to treat prostate cancer) proved that one could target the AR with molecules that weren’t traditional steroids. Flutamide and its 1990s successor, bicalutamide, showed that drugs could bind the androgen receptor with high receptor binding affinity yet have very different effects than testosterone (in their case, blocking the receptor in prostate cells). These developments hinted that chemical structure and binding mode mattered greatly for receptor behavior. By the end of the 1980s, all the pieces were in place: scientists had a growing understanding of receptor biology, evidence from SERMs that selective receptor modulation was possible, and new chemical tools to experiment with. This fertile scientific environment was the foundation of tissue selectivity becoming central to hormone therapy. It set the stage for the 1990s and beyond, when the first true selective androgen receptor modulators would finally emerge.
Leading scientists of the era helped drive this transformation. Figures like Dr. Elwood Jensen (who elucidated hormone receptors) provided the knowledge base. The pioneers of tamoxifen, such as Dr. V. Craig Jordan, demonstrated a practical example of selective therapy in action. And endocrinologists like Dr. Enrique Negro-Vilar heralded the idea of selective androgen modulators as the next big advancement – by 1999, Dr. Negro-Vilar was calling these compounds “a novel approach to androgen therapy for the new millennium.” In essence, the 1980s gave hormone therapy a new vision: targeted, tissue-specific action was not only feasible, but it represented the future of hormonal treatments.
Mechanisms Behind Tissue Selectivity in Hormone Therapy
How exactly can one drug act differently in different tissues? Several biological and pharmacological principles enable tissue selectivity in hormone therapy. Selective receptor modulators leverage a few key mechanisms to achieve their tissue-specific effects:
- Ligand-Induced Receptor Conformation: When a selective modulator (whether a SERM or SARM) binds to its hormone receptor, it induces a unique shape change in the receptor’s structure. This altered receptor conformation can expose or hide specific docking sites for other proteins inside the cell. As a result, the drug-bound receptor recruits a particular set of coactivator or corepressor proteins that is different from those recruited by the natural hormone. Each tissue has a different repertoire of these co-regulator proteins. For example, a certain SARM may twist the androgen receptor just enough to preferentially turn on muscle-growth genes, but not the genes that cause prostate cells to multiply. In this way, the same receptor can transmit a different signal depending on the drug’s shape and the tissue’s context.
- Partial Agonist/Antagonist Activity: Many selective receptor modulators act as full agonists in some tissues but only partial agonists (or even antagonists) in others. In muscle and bone, a SARM might fully activate the androgen receptor, leading to robust anabolic effects. But in tissues like the prostate or skin, that same SARM might only partially activate the receptor – essentially “dimming the switch” instead of turning it fully on. By providing just a weak signal in those sensitive tissues, the modulator can block the stronger natural hormones from binding (acting almost like a competitive inhibitor) without overstimulating the tissue. A classic analogy is tamoxifen’s behavior: it weakly activates estrogen receptors in bones and uterus (enough to have beneficial estrogen-like effects) while blocking estrogen receptors in breast tissue. Similarly, an ideal SARM can occupy androgen receptors in the prostate, without fully stimulating them, thereby protecting that tissue while still giving muscle cells a strong growth signal.
- Avoidance of Off-Target Metabolism: The chemical design of modern selective hormone therapies often sidesteps the metabolic pathways that amplify side effects. Take testosterone as an example – in certain tissues, enzymes convert testosterone into DHT or into estrogen, which can then cause unwanted effects (DHT strongly drives prostate growth and hair loss, while estrogen from testosterone can affect fat and breast tissue). Nonsteroidal SARMs are deliberately crafted so that they do not get converted by 5α-reductase or aromatase enzymes into these other potent hormones. They remain in a “fixed” form that only interacts with the androgen receptor in its intended way. By not generating high local DHT or extra estrogen, these selective drugs avoid triggering the secondary pathways that lead to prostate enlargement, acne, fluid retention, or breast tissue growth in men. This metabolic stability is a key reason SARMs tend to cause fewer traditional steroid-like side effects.
- Receptor Distribution and Co-Regulators: The expression level of receptors and the balance of co-regulator proteins can differ widely between tissues. Muscle and bone tissues typically have abundant androgen receptors and plenty of coactivator proteins that favor anabolic (building) processes. The prostate gland, on the other hand, while rich in androgen receptors, also contains different ratios of coactivators and corepressors. Some selective modulators may bind to AR in the prostate but, because the necessary coactivators aren’t as available or the modulator-receptor complex isn’t optimal for recruiting them, the downstream signal remains weak. Essentially, the intracellular environment acts as a filter. Muscle cells amplify the drug’s signal (leading to gene activation for growth), whereas prostate cells muffle the signal. This inherent difference in how tissues process the receptor’s activation contributes significantly to tissue selectivity – the same drug-receptor combination might be potent in one cell type and much less active in another.
- Pharmacokinetics and Tissue Distribution: The way a drug is absorbed, distributed, and broken down can also contribute to its tissue selectivity. Many selective hormone therapies are designed to be taken orally and circulate throughout the body, but their chemical properties might cause them to concentrate more in certain tissues. Some experimental SARMs, for instance, show higher uptake in muscle than in other organs. Others are formulated to have short half-lives, so they give brief pulses of activity. A shorter-acting drug might maintain continuous receptor engagement in muscle (if muscle cells absorb it readily) while giving other tissues periodic “breaks” as the drug levels ebb between doses. In practical terms, if a SARM tends to accumulate in muscle tissue, the muscles get a higher effective dose than the prostate or brain does. These pharmacokinetic tweaks – whether through molecular size, lipophilicity, or dosing schedule – help ensure the targeted hormonal effects are strongest where we want them and weaker elsewhere.
Through these mechanisms, modern hormone therapies turn receptors into precision tools. Instead of flipping every switch in every cell, a selective modulator fine-tunes which signals occur in which location. This multi-pronged selectivity strategy is what allows a drug to, say, build muscle without over-stimulating the prostate, or to treat breast cancer while actually strengthening bone. It’s a sophisticated interplay of chemistry and biology – truly a scientific breakthrough that has redefined what hormone therapy can do.
Clinical Benefits of Adopting Tissue Selectivity
Adopting tissue selectivity as a core design principle in hormone therapy has led to markedly improved clinical outcomes. The biggest benefit is a vastly improved therapeutic index – patients can achieve the desired therapeutic effects with far fewer side effects, which in turn means treatments are safer and often more effective. In traditional hormone therapies, side effects often limited how high a dose doctors could prescribe or how long a patient could stay on treatment. With selective receptor modulators, that calculus changes for the better.
Consider the development of SARMs for muscle-wasting conditions. Because these drugs selectively stimulate muscle and bone, patients can gain muscle mass and bone density without experiencing the host of androgenic side effects that come with anabolic steroids. In one clinical study, for example, a SARM called LGD-4033 (ligandrol) was given to healthy young men for several weeks. The results showed a significant increase in lean muscle mass and strength, but importantly, there was no significant change in prostate-specific antigen (PSA) levels – a key marker of prostate stimulation – and no signs of prostate enlargement or other androgenic problems. For comparison, a similar muscle gain from high-dose testosterone would almost certainly come with elevated PSA and prostate growth. Achieving muscle-building benefits without the prostate paying a price demonstrates a clear clinical advantage attributable to tissue selectivity. In practical terms, tissue-selective therapy means we can push for greater results (more muscle, better physical function) in patients who need it, without being stopped early by dangerous side effects.
Early trial results with other SARMs echo these findings. Studies in older adults have found that certain SARMs increase lean body mass and improve physical performance with minimal side effects, an outcome that traditional hormone therapy struggled to deliver due to safety concerns. Even in preclinical research, the pattern holds: in androgen-deficient animal models (like castrated rats), SARMs were able to restore muscle and bone growth nearly to normal levels while keeping prostates very small. In one remarkable experiment, a high dose SARM increased muscle size to well above normal in rats, yet the prostate gland remained at only a fraction of its normal weight. This kind of extreme separation – muscles responding robustly, prostate staying quiescent – highlights the therapeutic potential of selective modulators. It suggests that even at doses that would be unthinkable for steroids, these new drugs might avoid the organ damage and hormonal side effects that typically cap our use of anabolic agents.
The clinical benefits extend beyond just allowing higher dosing. Tissue selectivity opens the door to treating patient populations who were previously off-limits for hormone therapy. For instance, consider hormone therapy advancementsin managing muscle wasting (cachexia) in chronic illness. Testosterone can help counteract cachexia in diseases like cancer or kidney failure, but it’s rarely used because of systemic risks – the last thing an oncology patient needs is a surge of hormones that might stimulate tumor growth or cause other organ strain. A tissue-selective SARM, however, could be used to boost muscle in cancer patients without significantly affecting the rest of the body or the tumor itself. In fact, SARMs like enobosarm (ostarine) have been tested in clinical trials for cancer-related muscle loss and even in certain cancers, aiming to exploit the idea that activating the androgen receptor in muscle can preserve physical function, while any action on tumor tissue is minimized.
Another example is age-related sarcopenia, the gradual loss of muscle mass in older adults. Traditionally, giving an elderly person testosterone to combat frailty was a risky proposition – older men are more prone to prostate issues and cardiovascular side effects from androgens. But with SARMs showing tissue-selective effects, researchers have been exploring them as a sort of “exercise mimetic” for seniors: a medication that can increase muscle and bone strength without the typical hormone-related hazards. Early trials have indeed reported that older individuals on SARMs experienced muscle gains and better stair-climbing power with good tolerability, suggesting a viable future therapy for frailty.
Tissue selectivity has also improved outcomes for endocrine disorders like osteoporosis. We know androgens can help build bone density, but giving women or older men potent androgens is not acceptable due to side effects. Some of the first SARMs were actually developed with bone health in mind. In animal models of osteoporosis, SARMs increased bone mineral density and strength while also adding muscle mass – and they did so without requiring co-treatment with estrogen or causing the virilizing side effects that anabolic steroids would. This dual benefit – strengthening both bone and muscle – is unique and clinically valuable for fracture prevention and mobility, and it’s made possible only by tissue-selective drugs.
Even standard hormone replacement therapy for men (hypogonadism, or low testosterone levels) stands to benefit. Testosterone replacement via gels or injections is effective, but it must be carefully monitored for prostate enlargement, sleep apnea, blood thickening, and other issues. A SARM could potentially serve as an alternative to traditional testosterone replacement – one that improves mood, libido, muscle mass, and bone density in men with low hormone levels, but with less stimulation of the prostate or red blood cell production. In theory, this means a man could get the positive aspects of hormone therapy (feeling more energetic, stronger, improved quality of life) with a reduced need to worry about tipping into high risk for prostate disease or needing phlebotomy for high hematocrit.
It’s worth noting that tissue selectivity is equally crucial in contexts like women’s health. Anabolic steroids have generally been off-limits for women except in very limited cases because of virilizing side effects. But a tissue-selective androgen could provide benefits like muscle strengthening or treatment of certain conditions without those masculinizing impacts. One cutting-edge example is the exploration of SARMs for stress urinary incontinence in women – the idea is to strengthen pelvic floor muscles pharmacologically. Early investigations suggest a SARM might help with this female condition by building muscle where needed, without causing male-type hair growth or voice deepening. In oncology, too, tissue selectivity is making new therapies possible: some breast cancers have androgen receptors and respond to androgen signals. Researchers have tested SARMs like enobosarm in women with AR-positive breast cancer, aiming to slow tumor growth. In trials, enobosarm showed tumor suppression and even improved patients’ lean body mass, all with minimal side effects and no significant masculinization. Such outcomes simply would not be possible with traditional, non-selective androgen drugs.
In all these cases, the focused action of selective modulators is what makes these therapies feasible and attractive. By delivering targeted hormonal effects where they’re needed and avoiding other tissues, these drugs improve the balance of efficacy to side effects. Patients get more benefit with less risk. Doctors can consider hormone-based treatments for conditions they wouldn’t have treated with hormones before. And importantly, it allows chronic use of hormone therapy in a way that was previously impractical – for example, giving a muscle-wasting patient a daily anabolic agent for months on end, which could never be done safely with old steroids. As Dr. Shalender Bhasin and colleagues have noted, many experimental SARMs act as full agonists in muscle and bone but only partial agonists in the prostate, resulting in clinical benefits like muscle gain and bone protection without prostate enlargement. This is precisely what early endocrinologists dreamed of and what modern pharmacology has achieved: isolating the “good” effects of hormones from the “bad.” Tissue selectivity isn’t just a perk or a theoretical advantage – it is the core feature that allows these new hormone therapies to exist as a distinct and valuable class.
Current and Future Implications of Tissue Selectivity
The influence of tissue selectivity on modern endocrine and hormone-related therapies is profound and continuing to grow. Hormone therapy advancements in the last two decades have all leaned toward making treatments more selective. In current clinical practice and research, the lessons of SARMs and SERMs are being applied across the board. For example, scientists are now exploring selective modulators for other hormone systems: there are experimental selective thyroid hormone analogs that aim to lower cholesterol or aid weight loss without affecting heart rate or bone density (problems that broad-acting thyroid drugs cause). There are even attempts to develop tissue-specific insulin analogs for diabetes, to target certain metabolic pathways without causing excessive weight gain or low blood sugar episodes. The success of selective androgen and estrogen modulators has essentially created a template for therapeutic precision in endocrinology—maximize a drug’s action where it helps, minimize it where it harms.
Focusing on the androgen field, the current landscape includes numerous SARMs in various stages of clinical trials. While as of mid-2020s no SARM has yet been approved for general medical use, the clinical research is very active. The concept of tissue selectivity drives this research: every new SARM candidate is assessed for its anabolic (muscle/bone) activity versus its androgenic (other tissues) activity, with the goal being a wide separation between the two. This has led to a pipeline of drugs that, in trials, have shown exciting results for muscle wasting diseases, age-related frailty, osteoporosis, and even hormone replacement. For instance, one SARM is in late-stage trials for treating cachexia in cancer patients, and another is being studied for improving physical function in elderly hip fracture patients. These investigational therapies exist only because tissue selectivity made the risk–benefit profile favorable enough to consider hormone-like treatment in these vulnerable groups.
The future implications are equally exciting. We can expect ongoing innovations based on selective modulation. As scientists deepen their understanding of receptor structures and co-regulator interactions, they can design even more finely tuned molecules. Future selective modulators might achieve even greater specificity – perhaps drugs that not only distinguish between muscle and prostate, but even between subtypes of muscle fibers, or between different regions of the brain for neuroendocrine therapy. Gene therapy and advanced biologics might also take inspiration from the tissue selectivity concept, seeking to deliver hormonal signals only to intended cells. Essentially, hormone therapy is moving toward an era of pharmacological innovation defined by precision. This could transform how we treat endocrine disorders: imagine thyroid treatments that target only the liver, or cortisol analogs that act only in immune tissues and not the bones.
Another implication of tissue-selective therapy becoming the norm is the potential for safer long-term use of hormones. Conditions that require lifelong hormonal intervention – from growth hormone deficiencies to menopause management – could be managed with selective compounds that patients can take for years with minimal risk. The targeted therapymodel also means combination therapies become more feasible. For example, one could pair a muscle-specific SARM with a bone-specific analog and an immune-specific steroid, each working in its domain without interfering elsewhere, to comprehensively treat an aging-related syndrome. Such finesse in therapy was unthinkable decades ago.
Importantly, the rise of tissue selectivity is reshaping regulatory and ethical perspectives on hormone use. With safer profiles, there may be greater acceptance of using these drugs in chronic conditions or in populations like women and the elderly, who were previously largely excluded from anabolic therapies. Sports medicine and rehabilitation are also eyeing these developments (though any use in athletics raises doping concerns, the medical benefit for injury recovery is being studied).
In summary, tissue selectivity has steered hormone therapy into a new trajectory – one that emphasizes therapeutic precision and personalized action. As of today, the concept is firmly at the heart of research and development for endocrine drugs. Looking ahead, virtually all new hormone therapies are likely to incorporate selective design elements. This not only improves patient safety and outcomes now but also promises a future where hormonal treatments for a variety of diseases are both highly effective and tightly controlled in scope. The revolution that began with 1980s hormone research is still unfolding, and it holds the promise of hormone treatments that deliver powerful results with unparalleled safety.
FAQ
What does tissue selectivity mean in hormone therapy?
Tissue selectivity means that a hormone therapy or drug is designed to act on certain tissues while avoiding significant effects on others. In practice, the medication triggers its desired effects (such as muscle growth or bone strengthening) in target tissues but causes little to no activation of receptors in other, non-target tissues. For example, a tissue-selective androgen treatment might build up skeletal muscle and improve bone density without stimulating the prostate gland or skin. By exploiting differences in receptor shapes and co-regulator proteins in each tissue, scientists tailor these drugs to have tissue-specific effects. The result is a more focused therapy – essentially targeted hormonal effects where you need them, and fewer side effects elsewhere in the body.
Why was the discovery of tissue selectivity in the 1980s crucial for hormone therapy?
During the 1980s, researchers uncovered that the same hormone receptor could be made to behave differently in different tissues – a finding that was revolutionary for hormone therapy. A key example was the development of tamoxifen and the concept of Selective Estrogen Receptor Modulators (SERMs). Scientists observed that tamoxifen could block estrogen’s action in breast tissue (beneficial for treating breast cancer) while simultaneously acting like estrogen in bones (helping to maintain bone density). This dual action proved that a drug didn’t have to be an all-or-nothing agonist or antagonist; it could selectively modulate a receptor.
The coinage of the term “selective receptor modulator” in the 1980s marked a paradigm shift. It meant that future hormone therapies could be designed to target specific tissues, heralding a new era of safer and more effective treatments. Essentially, 1980s hormone research provided the first blueprint for how to separate a hormone’s helpful effects from its harmful ones, laying the foundation for today’s tissue-selective therapies like SARMs.
How has tissue selectivity improved clinical outcomes?
Tissue selectivity has improved clinical outcomes by making hormone-based treatments both safer and more effective. By minimizing unwanted hormonal side effects, patients can tolerate treatments at doses that achieve better results. For instance, selective estrogen modulators allow doctors to treat breast cancer or osteoporosis without damaging other tissues – tamoxifen can treat tumors while actually protecting bone health. Similarly, selective androgen receptor modulators can stimulate muscle and bone growth without causing prostate enlargement, hair loss, or liver toxicity. This means patients gain the benefits of hormone therapy (such as increased muscle strength, bone density, or improved vitality) without the trade-offs that used to limit those therapies.
In practical terms, a therapy that is tissue-selective can be continued longer or used in populations previously deemed too high-risk. The outcome is a higher quality of life and better disease management. Patients get significant improvements – like greater muscle mass, improved mobility, or stabilized bone structure – while avoiding complications that often interrupted or contraindicated traditional hormone treatments. This precision approach has validated itself in clinical studies, where tissue-selective drugs achieved therapeutic goals with few side effects, underscoring why they are seen as a major hormone therapy advancement.
Conclusion
Tissue selectivity has fundamentally reshaped the landscape of hormone therapy. By focusing on receptor specificity and tailoring drugs to engage only particular tissues, researchers have turned hormonal treatments from blunt instruments into precision tools. Nowhere is this evolution more evident than in the development of SARMs – molecules engineered to provide the anabolic benefits of testosterone with far fewer side effects. The ability to separate muscle-building actions from, say, prostate stimulation is not just a technical tweak; it represents a paradigm shift in how we approach endocrine treatments. Patients who need hormone therapy for conditions like muscle wasting, osteoporosis, or hypogonadism can look forward to treatments that are both effective and safe over the long term.
The impact of tissue selectivity reaches beyond just androgens. The same principle is being applied across other hormone systems, all with the goal of targeted hormonal signaling: maximize the drug’s action where it helps, and minimize it where it harms. In every case, the strategy is the same – turning endocrine therapy into a form of targeted therapy. We have truly entered an era of pharmacological precision in hormone therapy, and tissue selectivity lies at its core. Ongoing research continues to refine these selective approaches even further. New selective modulators and related compounds are in development, guided by ever-deepening knowledge of molecular biology and receptor dynamics. Understanding tissue selectivity is key to understanding the future of hormone treatments. It’s an exciting frontier where medicine is learning to harness the body’s signaling networks with exquisite control, delivering robust therapeutic benefits with minimal collateral effects. As research progresses, hormone therapy will only become more individualized and accurate – a promise that began with the revolutionary concept of tissue selectivity and continues to drive scientific breakthroughs in endocrine science.
About the author
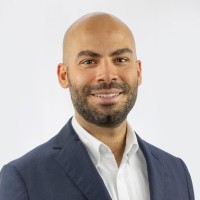
Emiliano Ventura, PhD, Pharm.D.
Senior Scientist MetID & LC-MS
🧑🔬 Senior Scientist | 🧪 Bioanalytical Methods (LC-MS/MS) | 🔍 MetID (HRMS, Radiodetection) | 💊 Small Molecules 🧱 Peptides 🧬 Oligonucleotides | 🚴 Anti-Doping | 🌍 Life Sciences | 🌟 Science with Purpose
Find me on LinkedIn!
Read more
Explore the Historical Development of SARMs in our series of articles:
Early Anabolic Steroid Era (1940s–1960s):
1. How the First Anabolic Steroids Were Created
2. Why Early Anabolic Steroids Fell Short of Medical Expectations
3. Hershberger Test: How Scientists Measured Anabolic vs Androgenic Effects
Discovery of Hormone Receptors (1960s–1970s)
1. How Discovery of Androgen Receptors Changed Hormone Therapy
2. Role of Nonsteroidal Antiandrogens in SARMs Development
3. The Crucial Difference Between Steroidal and Nonsteroidal Androgens
Concept of Selective Receptor Modulators (1980s)
1. The Revolutionary Concept Behind SERMs
2. Why SERMs Became a Blueprint for SARMs
3. How Tissue Selectivity Became the Core of Hormonal Drug Development
The Birth of SARMs (1990s)
1. The Story Behind the First Nonsteroidal Androgen Receptor Agonists
2. James Dalton’s Groundbreaking Research on SARMs
3. Early SARMs Structures and Their Advantages Over Steroids
Rapid SARMs Expansion (2000s)
1. Early Human Trials of Ostarine: Promises and Results
2. Ligandrol (LGD-4033): A Powerful SARM in Clinical Research
3. Chemical Diversity of SARMs Developed in the 2000s
Clinical Trials and Regulatory Challenges (2010s)
1. Phase III Clinical Trials of Ostarine: Successes and Failures
2. Why Defining Clinical Endpoints Was Difficult for SARMs Trials
3. Regulatory Hurdles Facing SARMs Approval
SARMs in Sports and the Rise of Misuse
1. How SARMs Became the New Doping Trend in Sports
2. Why Athletes Were Attracted to SARMs Despite Risks
3. Health Consequences of Illegal SARMs Use: Documented Cases
SARMs Today and Future Perspectives (2020s)
1. Current SARMs Research: Where Do We Stand?
2. Potential Medical Applications of SARMs in the Next Decade
3. Emerging Compounds and Future Directions in SARMs Research